While The Grow Monster enjoys growing plants in our suburban garden here on earth we also look up to the heavens and wonder what it would be like to have a suburban astrogarden up in space. This wonderment lead us to become familiar with a relatively new term (at least for us anyways) called ‘astrobotany‘.
So, what is astrobotany? Answer: It is basically the study of plants in space environments.
The Grow Monster will further describe what astrobotany is by exploring:
- Definition of Astrobotany
- History of Astrobotany
- Astrobotany Challenges
- Astrobotany and The Future
Definition of Astrobotany
As stated in the beginning, astrobotany is the study of plants in space environments. Astrobotany is a branch/discipline of study under Astrobiology. Astrobiology being the broader study of life and its possibilities in the universe around us. From the standpoint of human space exploration, astrobotany is the study of plants and their behavior in micro-gravity (some say ‘zero gravity’) space environments with the intention of growing them for food to sustain the astronaut’s nutrition requirements as well as provide for their psychological well being. This study also includes exploring the requirements of plant life and sustainment on other planets / celestial bodies in the universe.
History of Astrobotany
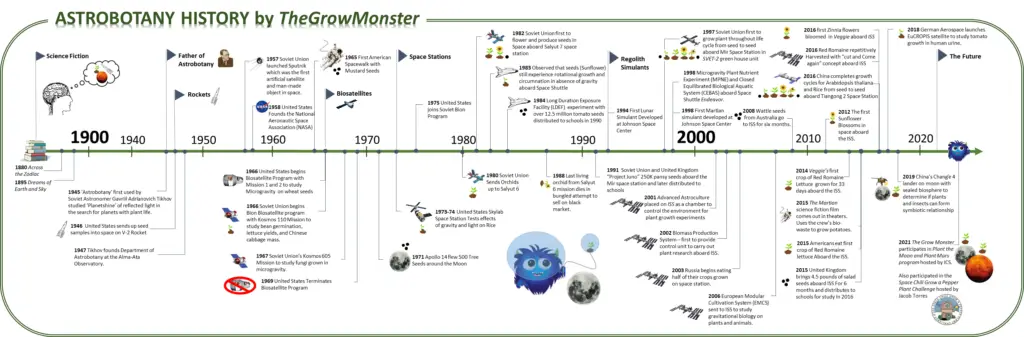
Science Fiction and Astrobotany
When it comes to understanding space and its possibilities, we often begin with science fiction where subjects could be freely explored as part of making great stories. For example, Star Trek has had a profound influence on the generations of scientists and engineers that came after. Who can argue that the flip-phone wasn’t partially inspired by this great work of fiction? It is these works of fiction that inspire readers to become scientists and engineers in those fields and make what was previously considered fiction into a reality. The earliest book touching on the subject of astrobotany (not the word itself) includes a novel written in 1880 called Across the Zodiac by novelist Percy Greg about a traveler who goes to Mars with plants. Then in 1895, Konstantin Tsiolkovsky – a Russian scientist – in his work entitled Grezy o Zemle i Nebe / Dreams of Earth and Sky, floated the idea of orbital greenhouses in space. One of the latest popular works of science fiction involving astrobotany includes the 2015 science fiction film called The Martian directed by Ridley Scott and starring Matt Damon. It was a screenplay adaption based on The Martian – a 2011 novel by Andy Weir. In this movie, Mark Watney -the mission’s botanist- is left behind to survive on Mars until his rescue. He creates a garden inside the crew’s Hab module using Martian soil amended with the crew’s bio-waste to grow unused potatoes left by the crew as well as utilizing left-over rocket fuel to create water. When The Grow Monster talks to family and friends about growing plants on Mars, The Martian movie seems to always pop up in the discussion.
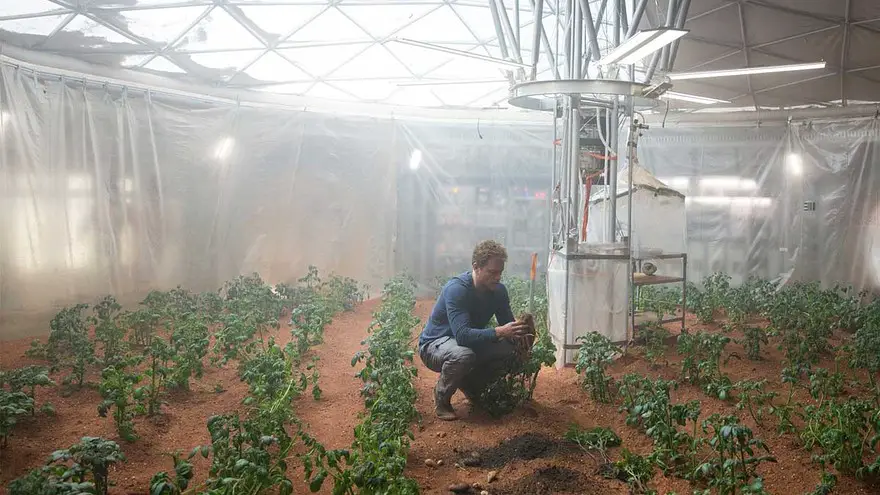
The Father of Astrobotany
The term ‘astrobotany‘ was first used by Soviet astronomer and astrobiology pioneer Gavriil Adrianovich Tikhov in 1945 and he is considered the father or astrobotany. Tikhov made the first attempts at detecting extraterrestrial vegetation by observing the light which a planet reflects. He coined the term ‘planetshine’. The idea was that planets with plant life utilizing photosynthetic pigments (e.g., chlorophyll) will have light spectrums in the range between 700-750 nm. In 1947, he went on to found the Department of Astrobotany at the Alma-Ata Observatory.
Rockets, Satellites, and Astrobotany
In order to better understand plants and their behaviors in micro-gravity, we needed a means of getting them into space. Upon the close of WWII, both the United States and Soviet Union had acquired the advanced Vergeltungswaffe / “Retribution Weapon 2” (V-2) rocket technology that the Germans had been pursuing. The United States acquired much of the people and teams that built the V-2 rocket including Von Braun along with other key people and teams that surrendered to the Americans after the war. The United States also acquired enough hardware to build 80 V-2 rockets. The Soviet Union, on the other hand, had acquired all of the manufacturing facilities and moved those back to the Soviet Union for further production. With a rocket technology to get seeds and plant life in space, the study of astrobotany could really take off (pardon the pun).
On July 9th 1946, the United States modified it’s recovered V-2 rockets to send up a number of seed samples into space, however those samples were never recovered. That launch was followed up with a second launch on July 30th 1946 to include a selection of maize seeds, which were recovered.
The space race began in 1957 when the Soviet Union launched Sputnik 1 (Earth’s first artificial satellite) into an elliptical low orbit around the Earth. This drove the need for the United States to coordinate it’s own space exploration with the establishment of the National Aeronautic Space Association (NASA) in 1958.
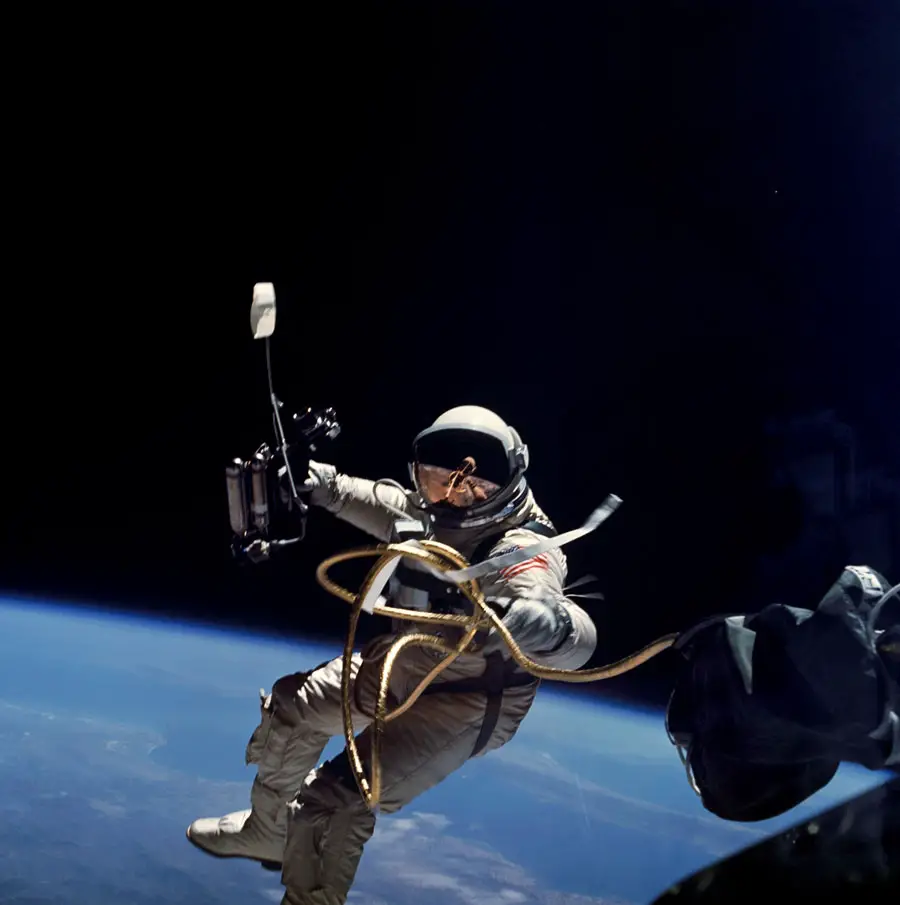
On June 3, 1965, the United States carried out it’s first space walk. During the Gemini 4 mission, astronaut Ed White had carried mustard seeds in his spacesuit’s pocket as a symbol of his faith. In Mathew 13 : 31-32, Jesus said in his parable, ““The kingdom of heaven is like a mustard seed that a person took and sowed in a field. It is the smallest of all the seeds, yet when full-grown it is the largest of plants. It becomes a large bush, and the ‘birds of the sky come and dwell in its branches.’ ” Mustard seeds are very small seeds that grow into very large plants. The seeds Ed White brought on the mission was an example showing that with just a tiny amount of faith, much can be accomplished.
The United States -through NASA- began a biosatellite program in 1966 with only three missions (Biosatellite 1, Biosatellite 2, and Biosatellite 3). Among other experiments aboard Biosatellite missions 1 and 2 was the study of the effects of weightlessness on wheat seedling morphogenesis and histochemistry, growth physiology, and the biochemical changes in the development of wheat seedlings in a weightless state. The United States terminated it’s biosatellite program in 1969.
The Soviet Union also began their biosatellite program called “Bion” in 1966 and continues to this day. With their first Kosmos 110 mission on February 22nd 1966, they sent up a number of plant species for 22 days in orbit. They observed that some bean varieties germinated poorly and that lettuce had 50% more yield. They also observed that a Chinese cabbage variety had a greater mass. In their second biosatellite mission, Kosmos 605 was launched in November 1967. What they observed was that fungi grown in micro-gravity created thin, bent legs with a larger mycelium than those found by the same species on earth. The United States was offered and accepted in 1975 to join the Soviet Union in their Bion satellite program after the United States had terminated it’s own biosatellite program in 1969.
In 1983 aboard Space Shuttle Columbia scientists wanted to study whether or not growth patterns of plants were innate or driven by the environment. Germination sunflower seeds while in orbit, they observed that seeds will still experience rotational growth and circumnation (growing in a spiral shape/pattern) even in the absence of gravity.
In April of 1984, Space Shuttle Challenger placed what was called the Long Duration Exposure Facility (LDEF) into a low Earth orbit. It was about the size of a school bus and the scientists’ intention was to study the long-term exposure and effects on space systems, materials, operations and the survival of selected fungal spores. Of the 57 experiments aboard the LDEF was also the popular Space Exposed Experiment Developed for Students also known as ‘SEEDS’. Over 12.5 million tomato seeds were placed on LDEF to test the affects of long-term exposure in space. The LDEF was intended to be retrieved March 19, 1985, but between scheduling delays and the Space Shuttle Challenger disaster (Jan. 1986), the facility was not retrieved from space until January 12, 1990 by Space Shuttle Columbia. Upon return to earth the seeds were distributed to elementary and graduate students alike with over 8,000 school reports written and provided back to NASA. What they found in the reports is that the space seeds germinated sooner, and grew at a faster rate than the control seeds. They also found that the seeds from space were more porous than those found on earth. Despite being in orbit for over five years, the viability of the seed still tended to be quite strong. School groups in the US and Canada can still participate in a similar program put on by the First the Seed Foundation called Tomatosphere.
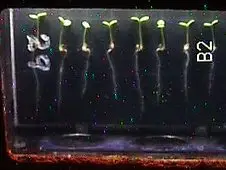
Around January 25, 1998 aboard Space Shuttle Endeavor during the STS-89 mission they conducted a hardware verification test that was called the Microgravity Plant Nutrient Experiment (MPNE). One of the biggest challenges they have experienced at this point with growing plants in microgravity is getting adequate levels of water and oxygen to the roots of plants. Because of the surface tension of water, they have observed either sever logging of water or an excessive drying around the roots in soil. With MPNE and it’s unique porous tubular design, they wanted to test the device to monitor and deliver the right quantities of water and nutrients to the plant roots as they needed them. Only after a few hours into the experiment, there was a failure in what was called the Water Availability Sensor (WAS). A backup system was implemented, however this resulted in the flooding of the wheat seeds that were used in the experiment.
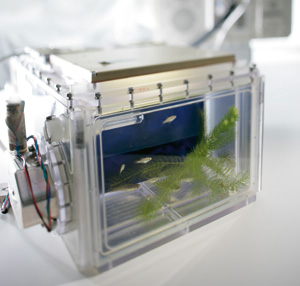
In addition to the MPNE experiment taking place aboard the Space Shuttle Endeavor during the STS-89 mission there was also the testing of a Closed Equilibrated Biological Aquatic System (CEBAS) minimodule. The CEBAS was a closed aquarium environment that was designed to sustain both plant and aquatic animals. The CEBAS minimodule included what was called a plant bioreactor. It was pre-loaded with 53 grams of a popular acquatic plant called Ceratophylium demersum (commonly known as hornwort, rigid hornwort,[1] coontail, or coon’s tail,). Aquatic animals were also placed into the environment that included pregnant fish, snails, and bacteria. A control group on Earth was also established with a 4 day delay. The system was given five days to adapt to it’s environment before being closed and placed on Space Shuttle Endeavor. Videos were taken of the plants and animal interactions every two hours for a ten minute period. The plant biomass had increased from 53 grams to 117 grams during the nine day duration of the experiment. The CEBAS experiment was considered the first successful flight of a closed self-sustaining aquatic ecosystem.
On December 3rd, 2018, the German Aerospace Center launched a low earth orbit satellite called the EuCROPIS, which contains two greenhouses used to simulate gravity conditions on both the Moon and Mars. The mission included growing tomatoes using human waste (urine) as a source for both moisture and nutrients (fixed nitrogen). Although much data was gathered during the mission, the mission itself failed due to a software problem. The mission ended on December 31st, 2019. The satellite is expected to orbit for the next twenty years before falling back to Earth.
Space Stations and Astrobotany
It wasn’t enough to just send rockets and satellites into space with seeds only to recover them to see if they grew any differently. A longer-termed solution was required to observe their life cycles end-to-end, and that solution was the creation of space stations. We humans needed a platform up in space in which plants can be grown and observed in a micro-gravity environment. No, we didn’t create space stations just for the sake of astrobotany, but they certainly helped promote the study of it. There have been 11 space stations launched into earth’s low orbit since 1971 with varying successes and not all of them supported astrobotany. A list of those includes: Salyut 1 (U.S.S.R), Cosmos 557 (U.S.S.R), Skylab (U.S), Salyuts 3, 4, 5, 6, and 7 (U.S.S.R), Mir, the International Space Station (U.S., Russia, multiple other countries), and Tiangong 1 and 2 (China). Those space stations supporting astrobotany experiments included Skylab, Salyut 6, Salyut 7, Mir (U.S.S.R/Russia), ISS, and Tiangong 2. More about these space stations can be learned here.
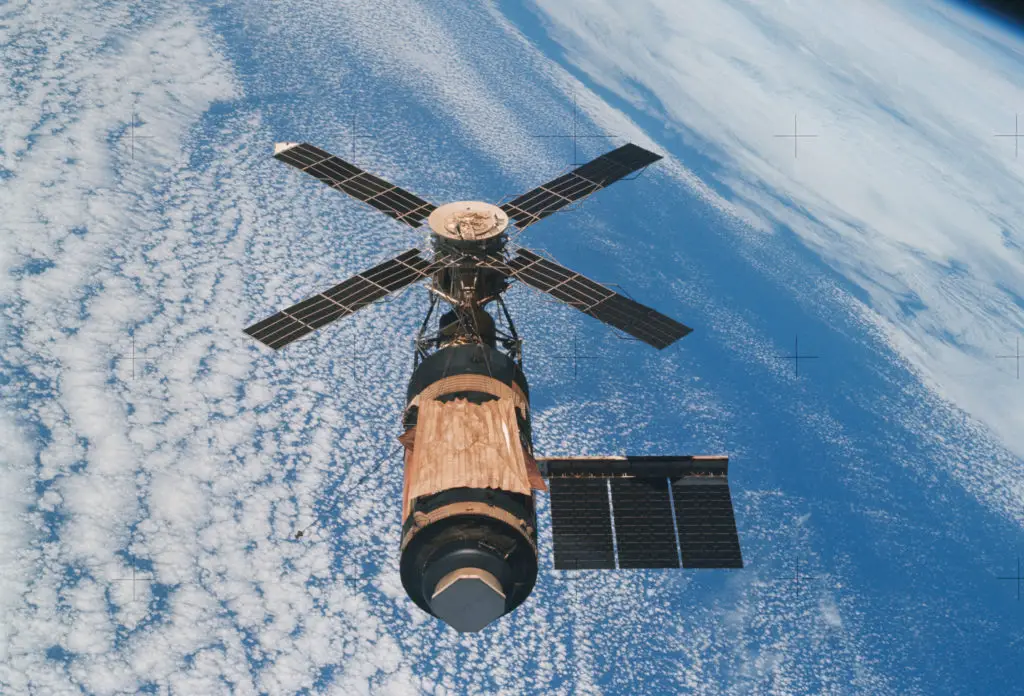
Skylab Astrobotany Experiments
Between 1973 and 1974, the United States had tested the effects of gravity and light on rice plants aboard it’s Skylab space station.
Salyut 6 Astrobotany Experiments
In 1980, the Soviet Union had sent up some orchid varieties aboard the Progress cargo craft for cosmonauts Leonid Popov and Valery Ryumin to care for. The experiment was primarily used to see if the plant interaction with the crew would boost their morale up in space. They also wanted to study the positive and negative gravitropism of orchid stems and roots. Understand that not all orchid stems grow upwards, and not all orchid roots grow downwards on earth. They found that growth rates for orchids slowed in space. Some of the varieties died in orbit. Now fast forward to 1988 with the last remaining orchid to have survived that mission. This orchid, named ‘Cosmonaut’, was stolen from the Academy of Sciences botanical garden in the Ukrainian capital of Kiev. According to an LA Times article, the police arrested a gardener named Vladimir Tyurin, age 36, who was using his access to the botanical gardens to steal and sell specimens on the black market. Unfortunately, the orchid had passed away in Tyurin’s bungled attempt to sell it. The article claims that ‘Cosmonaut’ was considered ‘priceless’ as it was still being studied in biological and genetic experiments as the only surviving orchid to have grown in a microgravity environment.
Salyut 7 Astrobotany Experiments
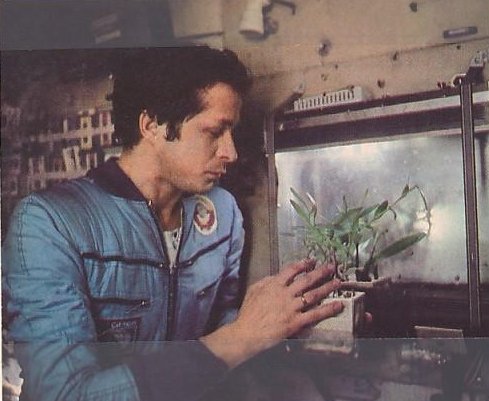
In 1982, the Soviet Union had been the first to successfully grow plants that flowered and produce seeds aboard it’s Salyut 7 space station.
Mir Astrobotany Experiments
On May 18 1991, the Soviet Union partnered with the United Kingdom on the Soyuz TM-12 mission. This was the 12th mission to the Mir space station. Aboard that mission was the first British cosmonaut in space, researcher Helen Sharman. The mission titled “Project Juno” was partially funded by private enterprise. This project included 250,000 pansy seeds placed into a Kvant-2 extravehicular activity (EVA) airlock. The Kvant-2 compartment is not as protected against the ionizing radiation as other parts of the space station and they wanted to study the affects that the cosmic radiation had on the seeds. Upon return, the pansy seeds were placed in packets of 25 and distributed to schools for study. The schools also received seeds in packets of 25 that were not in space to serve as the controls for the experiments.
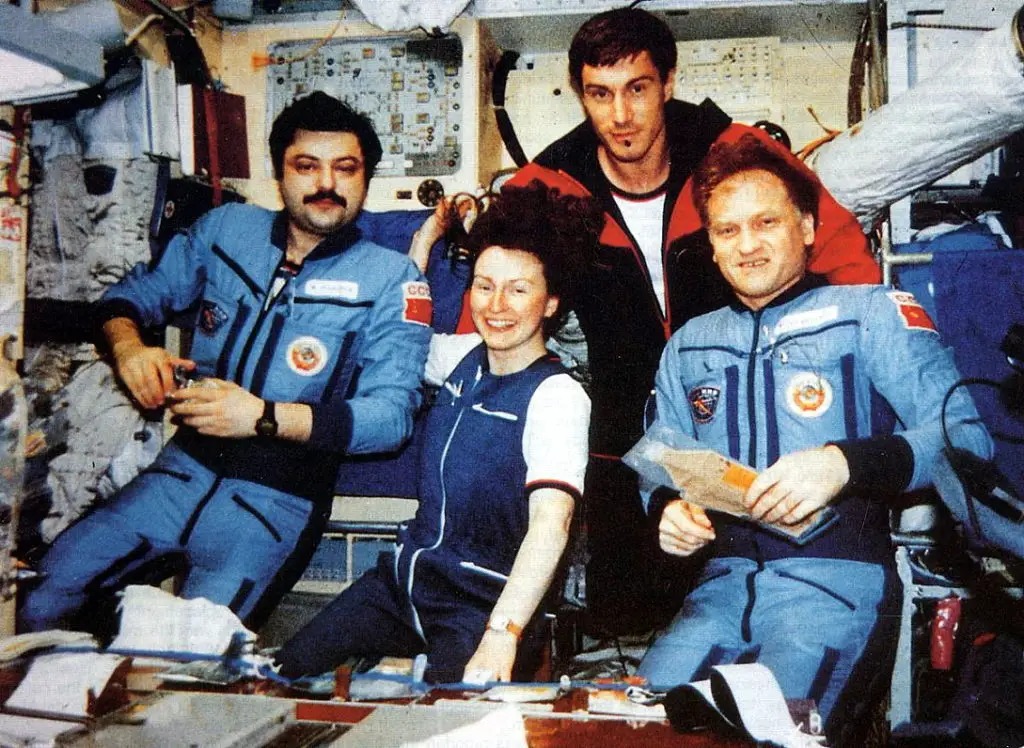
Then in 1997, the Soviet Union had been the first to successfully grow plants through the entire life cycle from seed to seed aboard their Mir space station using what they called the SVET-2 greenhouse unit. Those seed included maize (corn) and Daucus carota (wild carrot / bird’s nest / bishops lace).
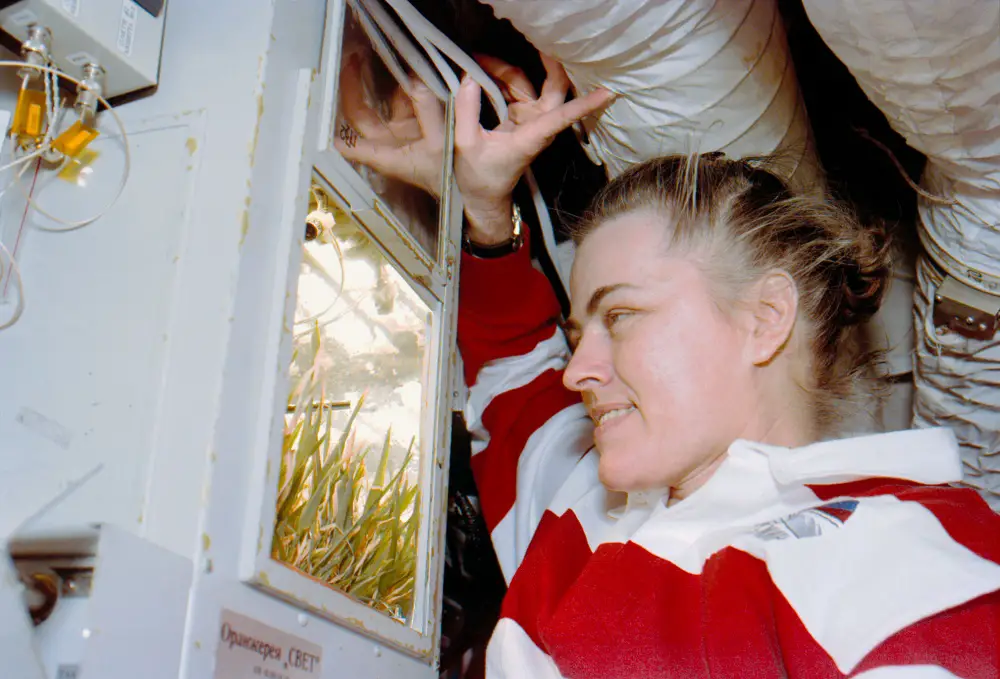
International Space Station (ISS) Astrobotany Experiments
Beginning in the mid 1990s, an opportunity for peace between The United States and Russia – among other countries-came with the co-creation of the International Space Station (ISS).
On March 10th, 2001, the Advanced Astroculture (ADVASC) unit was taken up to the ISS on Space Shuttle Discovery during Expedition 2. The ADVASC system was a commercially sponsored payload with the purpose of acting as a chamber for controlling the environment in which plants can be grown. It controlled all the parameters necessary for plant growth including temperature, relative humidity, light, delivery of fluid nutrients, carbon dioxide, and ethylene concentrations.
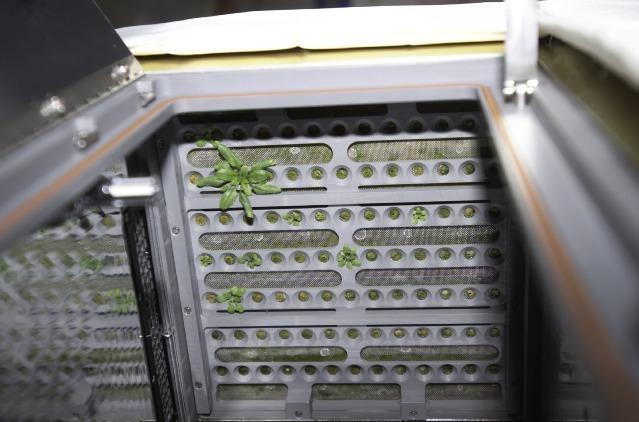
As part of the ADVASC experiment was the “Orbital Laboratory” program. This was one of the first student-designed experiments to be aboard the ISS. It included a kit for the school along with a multi-media education program available over the internet which provided students with the ability to design, conduct, and analyze the experiment taking place on the ISS. A student experiment database was established and upon completing the experiment on the ISS, students were able to recreate experiments virtually using experiment data from the student database.
The ADVASC conducted it’s first seed-to-seed experiment in space by completing the lifecycle of Arabidopsis thaliana and soybeans throughout their entire life cycles. The experiment’s purpose was to determine if plants can complete their seed-to-seed cycle in a microgravity environment, along with studying gene expression and chemical differences between seeds produced on earth versus seeds produced on the ISS. Upon completion of the experiment, they concluded that the Arabidopsis seeds were about half the size of those seeds grown in controls on the ground. The protein content and germination rates of the seeds are similar to those grown in the controls on the ground. They also observed that secondary branches and seed pods for Arabidopsis grew perpendicular to the main stems versus the upward growth seen in plants grown on Earth, which indicated that it was gravity -and not light- that had a role in the orientation of branches and pods. With the soybeans, they found that those grown in space were about 4% larger than those grown in ground controls. The quantities of seeds produced between space and ground controls were about the same however the seeds produced in space were larger than those produced in ground controls. The duration of the experiment and unit aboard the ISS lasted through Expedition 5 and was returned December 2002.
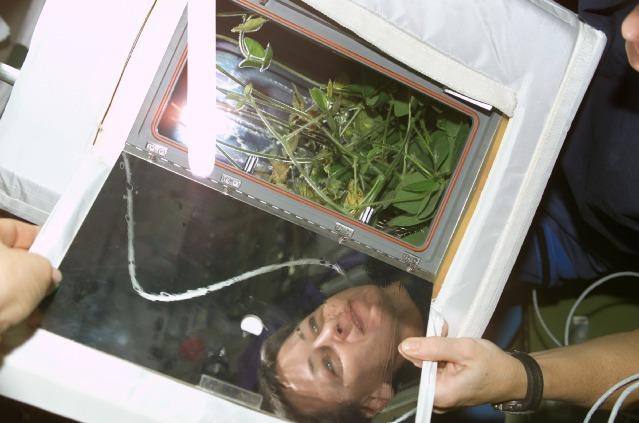
On April 8th 2002, the first Biomass Production System (BPS) was sent to the ISS during Expedition 4. The idea behind the BPS unit was to provide plant scientists with a customizable research environment aboard the ISS. The BPS is considered the first unit to provide the precise environmental controls necessary to carry out plant research in microgravity as it literally reports the conditions that the plants are living in by the minute. Two experiments took place in the BPS: the Technology Verification Test (TVT) and the Photosynthesis Experiment and System Testing and Operation (PESTO) experiments.
The TVT experiment simply validated the systems and protocols used to grow plants in microgravity environments. Brassica rapa plants were grown over two seed-to-seed cycles on the ISS. The growth of the Brassica between ground controls and those aboard the ISS were comparable, but there were differences in the chlorophyll, starch, and soluble carbohydrates concentrations of immature seeds. The concentrations of these were higher in those immature seeds grown on the space station. This suggests that microgravity may impact the flavor and nutritional quality of produce grown in space. They also determined that more fungal and bacteria specie communities were present in the BPS chambers in space than in those BPD chambers on the ground with an uncertainty in what contributed to the differences.
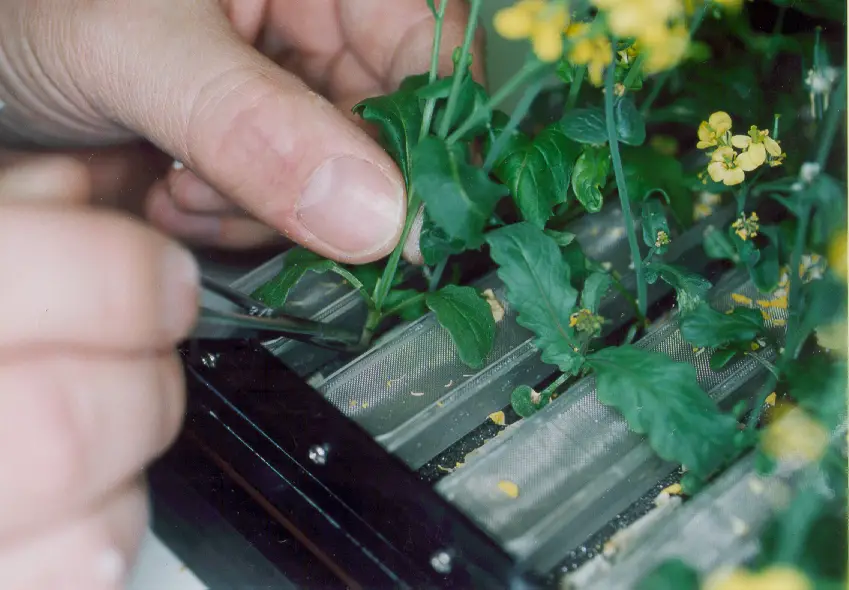
The PESTO experiment taking place in the BPS tested the photosynthesis and metabolism of wheat plants (Triticum aesativum). They observed high germination rates with leaf growth being comparably the same between those grown on Earth and those grown aboard the ISS. They also determined that microgravity is not a major stressor to the plants. The only difference being that leaf cross-sections were different as those grown in microgravity tended to have a thinner cross-section. They also noted a structural difference in the space-grown chloroplasts being more oval in shape versus those grown on earth. They found no differences between the plants when it came to soluble sugars, lignin content in leaves, starches, or gene expressions.
Both of the 73-day BPS experiments together concluded with eight harvests, 300 plant tissue samples in archive, 3000 images of plants grown throughout the cycles, along with a complete set of BPS hardware performance data for analysis.
What The Grow Monster would like to determine is the difference between the ADVASC and the BPS systems. Did the ADVASC experiment lead to the development of the BPS, but with a name change? Or did ADVASC become a component of the BPS? Or was BPS a component of ADVASC, or were they just two projects taking place in parallel? If anybody has more information, please leave in the comments.
In July 2006, the European Modular Cultivation System (EMCS) was also sent to the ISS to study gravitational biology of not only plants, but on insects, amphibians, invertebrates and tissue cultures.
On May 31st, 2008 the Space Shuttle Discovery included a number of Australian Golden Wattle seeds for study by Dr. Gregory Chamitoff. They were aboard the ISS for about six months before returning to Earth. There is a particular interest in studying Wattle seeds with their rapid growth abilities including the ability to enhance the soil conditions around it, reduce compaction, and paving the way for other plants to grow. In 2014, some of the seeds that returned from this mission were grown into tress and planted in Sydney Australia’s Royal Botanic Garden. In October of 2020, Wattle seeds were scheduled again to go up to the ISS aboard SpaceX 21 mission for six months as part of the Australian Seeds in Space education program where students will be able to plant the seeds for study. This was sponsored by the One Giant Leap Australia Foundation.
The ISS became fully operational in May 2009.
The BPS technology and experience gained over the years has led to the development of what is now called a Vegetable Production System (Veggie). On May 8th, 2014 with Expedition 40, Veggie had started it’s first crop of ‘Outredgeous’ Red Romaine lettuce growing for 33 days with the VEG-01A experiment. Although the Russians have been eating half their crops and saving half for research in space since 2003, Americans started eating their Red Romaine harvest during Expedition 44 on August 10th, 2015 with the VEG-01B experiment. Other interesting things that have blossomed in the space station include a sunflower in 2012, along with a zinnia in 2016 during the VEG-01C experiment. They began experimenting with the concept of “cut-and-come-again” where the Red Romaine was repetitively harvested during the VEG-03A experiment between October 25th, 2016 and December 28th, 2016. They tested this concept again but with ‘Tokoyo Benana’ Chinese Cabbage between April 3, 2017 and May 31, 2017.
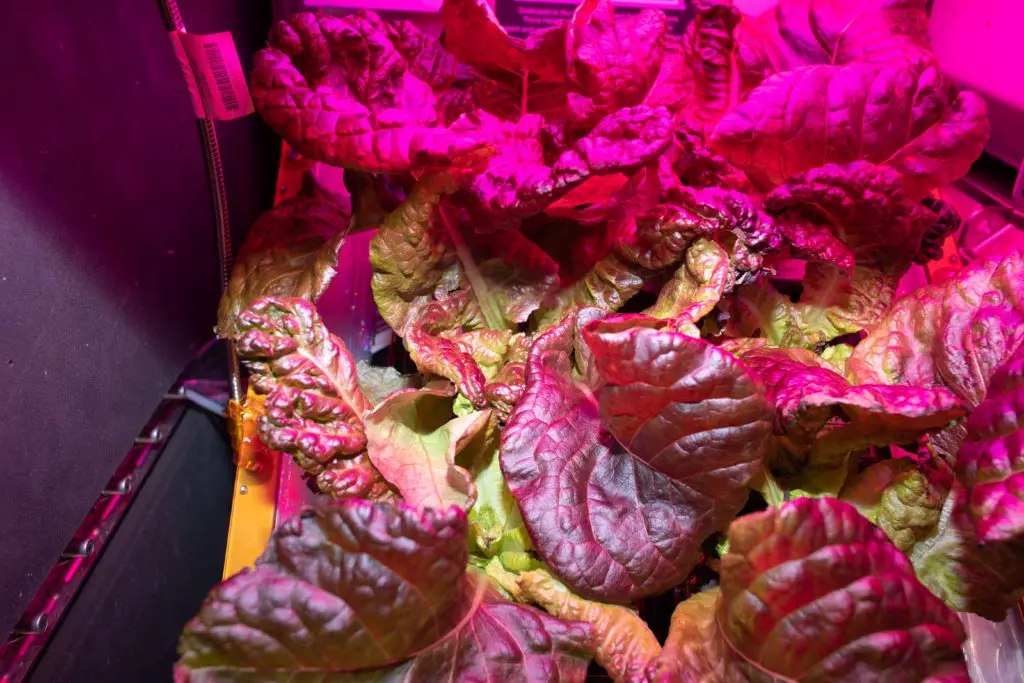
In 2015, British astronaut Tim Peake with the European Space Agency (ESA) took nearly four and a half pounds of salad seeds (Eruca sativa) with him on his six month mission aboard the ISS. When the seeds returned to earth in 2016, they were distributed to about 600,000 school children across the United Kingdom to germinate and grow to see if space radiation (up to 100 times more powerful than Earth) would impact the germination of the seed. What they found is the ‘rocket’ salad seeds grew at a slightly slower rate than those that remained earth bound. From this, they suspect that spaceflight reduced the ‘vigor’ of the seeds and accelerated the ageing process.
On January 16th, 2016, a Zinnia became the first flower to grow and bloom in the Veggie system. The experiment began on November 16th 2015 when the Veggie system was activated with Zinnia seeds embedded into what were called “rooting pillows”. However, in late December, the growth of the Zinnia’s were not looking so good as they were wilting and required more water. The bureaucracy and protocols in place were not accounting for additional water requirements for the plants, so it was determined that the astronauts caring for the plants should be given some flexibility in exercising their best judgement on when the Zinnias should be watered. The Veggie team had published a simpler guide called “The Zinnia Care Guide for the On-Orbit Gardener”.
In 2017 came the Advanced Plant Habitat (APH) design for the ISS. The main difference between the APH and Veggie is that APH is more self-sustaining and requires less human involvement. In 2018, they began using plant pillows and root mats in support of growing food for the crew.
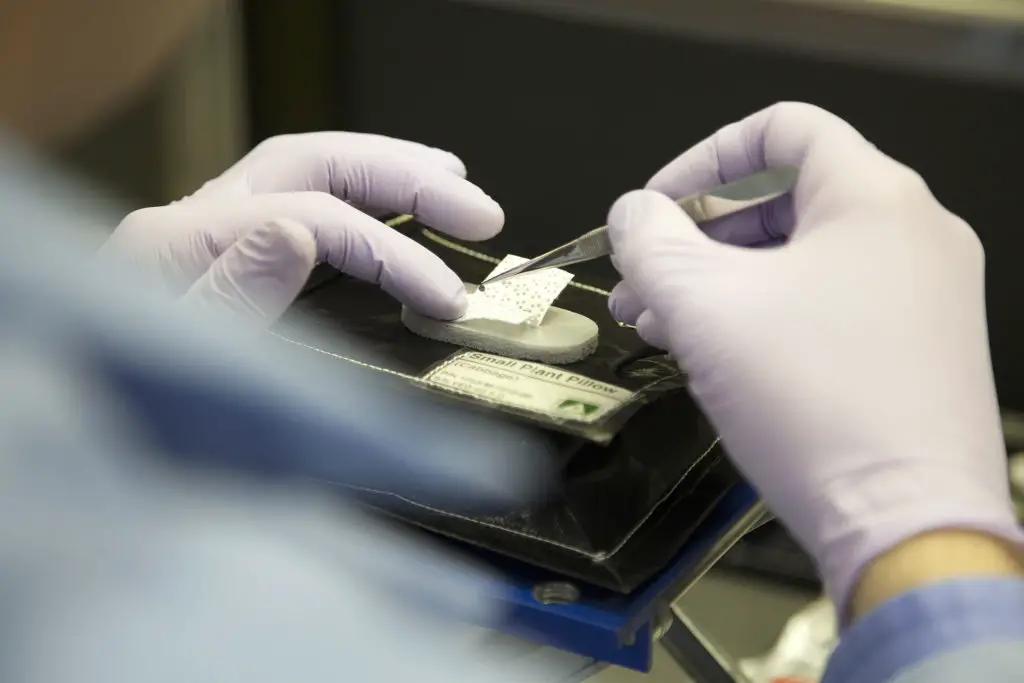
Tiangong 2 Astrobotany Experiments
The Tiangong 2 space station was launched September 15th 2016 and by November 2016, China was able to complete their first long-term plant experiment in space by successfully completing the growth cycles for both Arabidopsis thaliana and rice from seed to seed . This was a first for Chinese scientists. They understand the necessity of being able to grow grains and vegetables in space if humans are expected to have long-term missions in space. With their experiments, they found that plants are slower-growing in space and will bloom much later. They also found that plants generally live longer in space as the Arabidopsis had survived twice as long as the Arabidopsis plant grown on earth, which has Chinese scientists looking at changes in genes that are possibly associated with age. The Tiangong 2 space station had a planned de-orbit and decommissioning on July 19th 2019.
Lunar Missions, Mars Missions, and Astrobotany
The Apollo 14 mission in 1971 included the flying of some 500 tree seeds around the moon with the intention of studying their growth upon returning to earth. Scientists were particularly interested in the effects that radiation exposure had on living tissues. No noticeable changes in growth were detected between those seeds flown around the moon and those seeds acting as a control on earth. These seeds included Douglas fir, Redwood, Sycamore, Sweetgum, and Loblolly pine. However, scientists are interested in locating any seedlings that have been passed out and went on to grow into trees. They refer to these as “Moon trees”.
On January 3rd 2019, China’s Chang’e 4 lunar lander mission landed on the moon with a sealed biosphere that set out to determine if plants and insects can form a synergistic relationship. The idea was that as the insects in the biosphere grew, they were to release carbon dioxide while the plants -in turn- provided the insects with food via growth and oxygen via photosynthesis. Without further research, The Grow Monster is not certain which insects were placed in the container as one source mentions ‘silkworm eggs’ and another source mentions ‘fruit fly eggs’, but the other plants mentioned in the articles include: Arabidopsis, potato seeds, rapeseed, and cottonseed. The experiment terminated January 16th 2019 as they were unable to maintain the temperature in the biosphere once the Lunar night had set in.
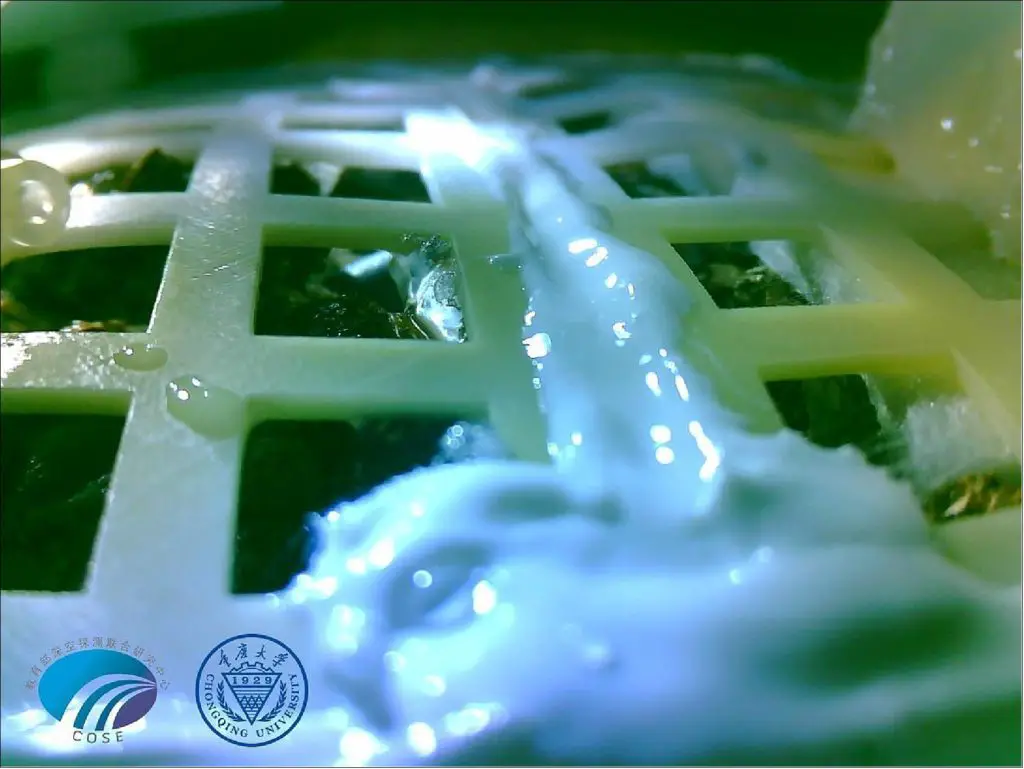
Regolith Simulants and Astrobotany
In 1994, the first lunar simulant (a simulated Lunar soil) was developed at NASA’s Johnson Space Center (JSC) and referred to this simulant as JSC-1. This simulant was based on data and actual lunar samples received from the Apollo missions. It was the first attempt at simulating those soils found in the Lunar Maria (Latin for “Seas”), which are the large, dark, basaltic plains on Earth‘s Moon formed as a result of ancient volcanic eruptions. In 2005, NASA contracted with Orbital Technologies Corporation (ORBITEC) to produce three grades of simulants including JSC-1AF, JSC-1A, and JSC-1AC. Each having differences in grain size and coarseness.
In 1998, the first Martian regolith simulant (a simulated Martian soil) was created. This simulant was also created at NASA’s Johnson Space Center (JSC) and was referred to as JSC Mars-1. They also created another version of the simulant called JSC Mars-1a. These were based on data collected during the 1976 Viking 1 and Viking 2 missions, along with additional data received from the 1997 Mars Pathfinder mission using the Sojourner rover (the first wheeled vehicle to rove on another planet). In 2007, the Mojave Mars Simulant (MMS) was developed to address issues with JSC Mars-1 and 1a. In 2018, the recipe was later perfected into what was next referred to as (Mars Global Simulant) MGS-1 to be the first mineralogically accurate Martian regolith simulant.
Astrobotany Challenges
There are two main challenges to growing plants in space: microgravity and ionizing radiation with oxidative stresses (free radicals). As humans have experienced, the affects of spaceflight on the body include a reduction in muscle mass, bone density, taste, and vision. Spaceflight also contributes to blood pressure issues. Plants also experience changes in their growth due to microgravity and ionizing radiation with oxidative stresses.
Microgravity
It is not that there is ‘zero gravity’ up in space, it is just that there is ‘less’ gravity the farther away you move from a large celestial body such as the Earth. If you’ve ever run water slowly from the tap and placed your finger next to the flowing stream, the water tends to bend toward the finger. It is not that your finger is exerting more gravity, but the flow of the water (due to surface tension and cohesion) towards the finger is similar to how gravity behaves between objects. Objects exert more gravity on one another the closer they are. Plants react to the presence or lack of gravity in what is called gravitropism. The roots of a plant tend to grow toward gravitational pulls (positive gravitropism) and stems tend to grow away from gravitational pulls (negative gravitropism). They’ve found that plants growing in microgravity tend to have shorter roots / root hairs, weaker cell wall structures, and that the direction of root growth can also be influenced by the light source (phototropism). If microgravity is impacting root growth with weaker cell wall structures, it will certainly pose a challenge for getting nutrients into the plant and establishing a structure that can grow and maintain a fruit if humans are to use them as a food source in space.
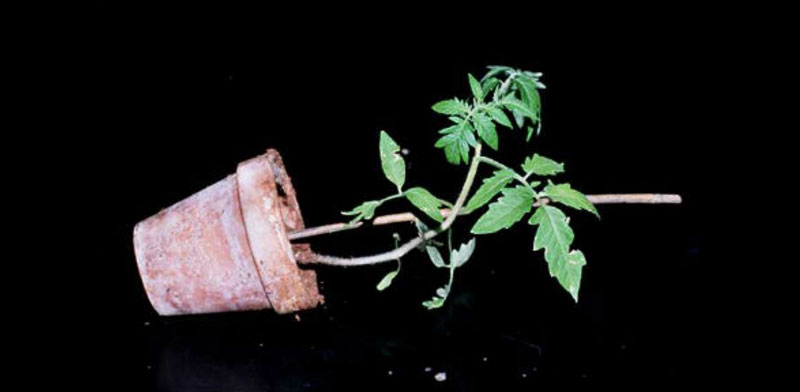
Ionizing Radiation with Oxidative Stress
You may not entirely appreciate earth until you have to leave it and its protection. Due to Earth’s size, it has a very large magnetic field that protects all life from what we call ‘ionizing radiation’. According to the Centers for Disease Control and Prevention (CDC), ionizing radiation is a form of energy that will act to remove electrons from both atoms and molecules of materials including air, water, and living tissues. Examples of ionizing radiation include x-rays, alpha rays, beta rays, and gamma rays that are accelerated to the speed of light from stars such as the sun (see chart from the CDC below). Ionizing radiation passes through these materials which means they have the capability to alter molecules within the cells of living organisms that increase what are called Reactive Oxygen Species (ROS) /free radicals in living tissues and can result in what is known as Oxidative Stress and Damage (OSaD). This may lead to cancer and mutations which pose a real health problem to living organisms in space. Understand that space ships are small in comparison to the earth and don’t have the kind of magnetic shield that earth has to protect against ionizing radiation. When it comes to astrobotany, ionizing radiation can influence the long-term growth of plants on journeys between larger celestial bodies such as the Earth and Mars, where there might be some protection against ionizing radiation. Plants could mutate favorably or unfavorably and more frequently with each seed-to-seed cycle on these trips. Until longer-termed experiments are conducted, we won’t entirely know the full impact that ionizing radiation will have on plant generations in space. So ionizing radiation poses yet another unpredictable challenge to growing plant life in space.
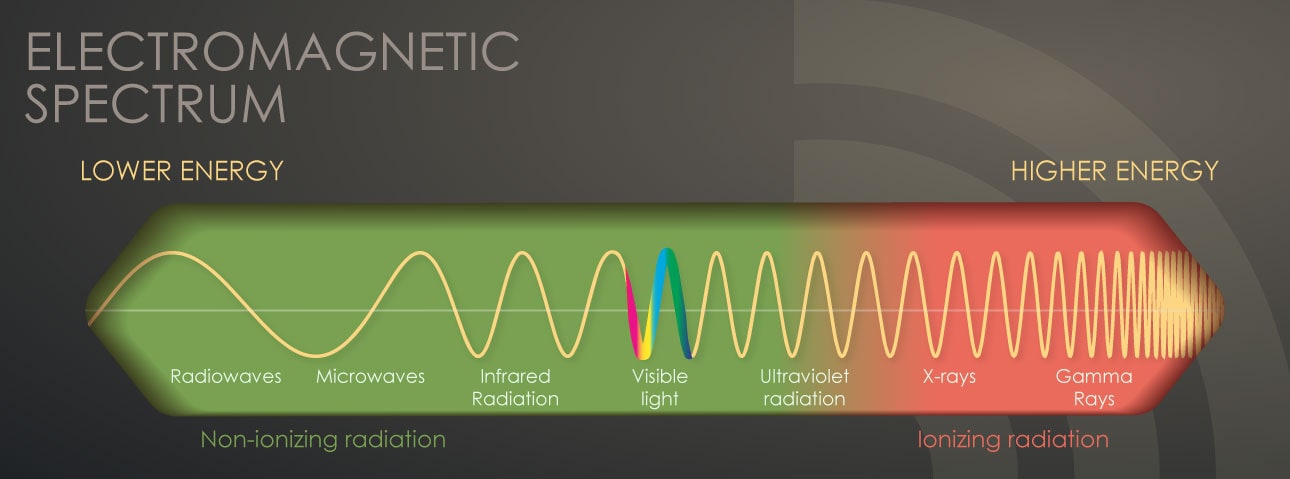
Other Challenges
There are a number of other important challenges in addition to microgravity and ionizing radiation with oxidative stresses (free radicals) that need to be considered when it comes to astrobotany. There is the carbon dioxide and oxygen gas exchange requirement for plants. Depending on the quantity of plants grown, astronauts may not produce all the carbon dioxide required for the plants to grow and systems will need to be in place to monitor the balance of carbon dioxide gases consumed and oxygen gases produced by the plants. There is also the challenge of insect and microbial life support in the soils being used for the plants. It can not be overstated how important bugs and smaller microbial life in the plant soil (e.g., bacteria, actinomycetes, protozoa, nematodes, and fungi) are for the growth of plants. These organisms and their processes help convert nutrients and make them available to the plant roots and even provide antibiotic properties for the plant. In return, the plant roots provide a source of food for these organisms in the soil in a symbiotic relationship.
Significant studies need to be made to determine how bugs and microorganisms behave in soil that simulates the Lunar regolith (Moon soil) and Martian regolith (Mars soil). They call lab-created test soils a ‘simulant’ where certain minerals found on earth are ground up to simulate those same mineral combinations located in various areas of places such as on the Moon and Mars. Seeds along with the microbes can be added to the simulant soil to observe how well they grow together and to determine the optimal combination of microbes needed to support plant life in that simulant. There are currently a number of labs in the US that produce this simulant like the Exolith Lab at the University of Central Florida.
Another significant challenge to astrobotany and the growth of fruit-producing plants is pollinator support. Some plants are self-pollinators (e.g., peppers, green beans, tomatoes), but other plants (e.g., squash, cucumber, pumpkin) require outside help from pollinators such as birds, bees, beetles, ants and others creatures that will typically go from flower to flower. Current experiments conducted in space have include manual pollination by the scientists working the experiment with small quantities of flowering plants, but as the requirement to produce mass quantities of produce in space increases, so does the requirement to address the need for pollinator support. Studies will need to be conducted to determine if pollinator insects, such as bees, can learn (or be trained) to navigate in a microgravity environment to do the work necessary to pollinate flowers. Studies also need to be conducted to determine as to what extent can bees navigate outside of Earth’s magnetic field along with orienting themselves to the artificial sunlight provided for the plants. In the mean time, other pollinator solutions will need to be pursued to meet the requirement such as robotic arm pollination or pollination by drones that go from one flower to the next up and down the line.
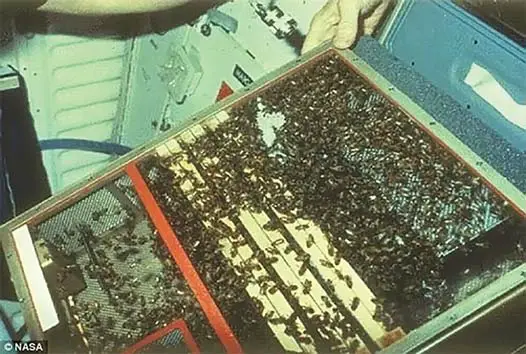
Astrobotany and the Future
As our understanding of astrobotany evolves, so will our practice of astro-horticulture, which would be the art and science of the cultivation of plants in space. The study of astrobotany and astro-horticulture will also lead -to a greater extent- to the practice of astro-agriculture, which would be the art and science of cultivating both plant and animals for the purposes of food, medicinal, aesthetic gratification, and other products (wait for it) in space! The Grow Monster is looking at this from a astro-horticulture / astro-agriculture point of view as we prepare to have a suburban astrogarden, along with our astrohome to include a beautiful astrolandscape with an astrocompost pile tucked away in the corner of our astroproperty. The Grow Monster envisions all this as part of its suburban astro-realestate investment up in space. However, none of this will be able to happen until mankind better understands what affects plants in space with our continued studies in the field of astrobotany.
Pingback: Plant the Moon and Plant Mars Challenge with NASA - The Grow Monster
Pingback: Anonymous